Great Neapolitan Earthquake of 1857/Part I. Ch. II
CHAPTER II.
ELASTIC WAVE OF SHOCK.
The elastic or earth wave of shock, may reach a given point upon the surface, with any angle of emergence (the angle contained by the horizontal plane with the wave-path at the point of emergence), or in any azimuth. The path of the wave is a right line joining that point with the centre of impulse (or focus), the wave being assumed propagated thence in all directions outwards in spherical shells. This is strictly true only in an homogeneous elastic solid.
Every point in a coseismal line (that in which such a wave shell simultaneously reaches the earth's surface) at the moment of shock describes a closed curve in space, returning to (or almost exactly to) the point from which it started into motion. The curve is one of double curvature, the vibration taking place nearly simultaneously, in three rectangular unequal axes. For the purposes of our inquiry we may neglect the transversal vibration, and may consider the closed curve of normal vibration as confined to vertical planes passing through the centre of impulse. In fact, the movement of the wave particles may be assumed as confined to right lines, indirectum with the path of the wave, whose length is equal to the amplitude of the wave or to one half of its complete vibration.
As the coseismal curve (or crest of a wave of shock) enlarges its area, travelling outwards in all directions from the seismic vertical—that is, from the vertical line passing through the earth's surface (and centre) and the focus—every point in and upon the surface, in succession moves once forward and back in the direction of the wave-path, and to the extent of its amplitude at that point, or in two components, vertical and horizontal, that shall give such direction.
We are not concerned here to consider the extent or the laws according to which the range of wave movement of each material particle, either in amplitude or attitude, or the velocity of its particles, vary with the distance from the focus.
The angle of emergence for any given depth of focus diminishes as a function of the distance of any given point of the surface from the seismic vertical; and I have shown elsewhere that the power of the shock to overthrow objects is, cæteris paribus, a maximum at a determinate distance all round the seismic vertical; and this distance would be equal all round, were the earth homogeneous, and the focus or centre of impulse confined to a mathematical point. The centre of impulse in nature, however, occupies determinate, and often large dimensions. For this reason as well as from non-homogeneity amongst others, neither the meizoseismic curve (or that of maximum overthrow) nor the isoseismic curves (or those of equal overthrow) are found to be circles or even perfectly regular closed curves, nor concentric.
For the same reason the observed angles of emergence will be found to vary from those that would be due to a focus of evanescent magnitude.
The distinction must be clearly borne in mind between the velocity of transit of the wave—that with which the advancing form or seismal curve is transferred from point to point of the surface, and that of the earth particles moving within the limits of amplitude of each vibration. The former velocity is very great, nearly half as rapid as that of a cannon shot, and depends chiefly upon the elastic modulus of the earth's formations through which the wave transit is made; but the latter, as now measured for the first time, is very small indeed, often not greater than that which a body acquires by falling from a height of two or three feet.
It is, however, to the rapidity of transit velocity, or, which is the same thing, to the great rapidity with which the proper velocity of vibration of the wave passes, from 0 to its maximum velocity, on reaching any material object, that the formidable dislocating effects of the very moderate maximum velocity of vibration are due. We need not, however, here extend these preliminary remarks.
The evidences fitted for observation after the shock, by which the conditions of earthquake motion are discoverable, may be divided into two great classes:—
1st. Fractures or dislocations (chiefly in the masonry of buildings) which afford two principal sources and sorts of information.
a. Information from the observed directions of fractures or fissures, by which the wave-path, and frequently the angle of emergence may be immediately inferred.
b. Information from the preceding united with known conditions as to the strength of materials to resist fracture, by which the velocity of the fracturing impulse may be calculated.
2nd. The overthrow, or the projection or both, of bodies, large or small, simple or complex. From these we are enabled to infer—
c. By direct observation the direction in azimuth of the wave-path.
d. By measurements of the horizontal and vertical distances of overthrow or of projection, to infer the velocity of projection and angle of emergence—both, or either.
Fractures or dislocations present themselves always in directions more or less transverse to the wave-path. Overthrow or projection, on the contrary, always takes place in the line of the wave-path, or in the vertical plane passing through it; but the direction of fall or of projection may be reverse (or in the contrary direction) to that of the wave transit, or it may be in the same direction with it.
At the moment of the arrival of the earth wave at any object upon the surface, whose dimensions are less than the amplitude—an obelisk or pillar or single wall for example—motion is suddenly communicated to the body; the velocity of the vibrating particles rapidly increases from zero to its maximum velocity, and returns to zero, as it completes its first semiphase or half-vibration, the direction of movement in which is in the same sense as that of the wave transit. With nearly the same rapidity the velocity increases in the opposite direction from zero to the maximum, and back to zero again. The wave has then passed the given point, its whole phase or entire vibration has been completed, and it has produced its effects. The movement applied, is opposed by the inertia of the body moved, whose motions and final displacement depend upon the direction of the wave-path with regard to the centre of the body, its form, and the position of its base, or points of adherence or of support, and to the maximum velocity of the wave's proper motion. The applied velocity acts at the centre of gravity and in the direction of the wavepath, and the body, if free, apparently moves in the opposite direction to the wave in its first semiphase in consequence of its inertia. The force of displacement, with a given maximum velocity of vibration is therefore always proportionate to M, the mass, so that a heavy body, in the same shape and conditions is as easily upset as a light one.
If the body be not free, if the line of wave transit passing through its centre of gravity pass within the base or through any other support, it does not move in the first semiphase of the wave; but if it be free in the opposite direction, it will be displaced in the second semiphase of the wave; but as the wave movement is now reverse to that of its transit, the inertia of the body acting still contrary to the applied velocity, now impels the body in the same direction as the wave transit.
In either case, and in either semiphase of the wave; the movement impressed, may be one of mere overthrow or upsetting, or it may be one of actual projection, or of both combined, depending upon the special conditions of the body and its supports, &c.
Where the body is projected from a base or support with which it has had friction or adherence, and that the line of wave transit through its centre of gravity does not also pass through the centre of adherence (that is, the point of the base, or between it and supports, in which all the resisting forces, of adherence, &c., may be supposed concentrated), then, besides projection, a movement round a centre of spontaneous rotation within the body will also be impressed. Where this is due to adherence at the base, the rotation is generally in a vertical plane, and does not seriously disturb the plane of projection from that of the wave-path, i.e., of a vertical plane passing through the seismic focus and the body displaced; but when also due to lateral adherence, or other still more complex conditions, the body is flung forward and whirls round on inclined axes, and finally comes to rest in some position quite abnormal to its original status, giving rise occasionally to complex phenomena from which nothing can be inferred. Where the body is large, such as a house or church of masonry, or even a single wall exposed to shock in the plane of its length, overthrow may be impossible with given dimensions and given angle of emergence of the wave; but in such case dislocation or fissuring occurs, and the severed parts may or may not be overthrown, dependent upon the amount of the applied velocity consumed in producing fracture only.
There may be no displacement whatever of loose objects, nor any dislocation of large masses, such as churches, &c., though exposed to violent shock, if its emergence be quite or very nearly vertical, and that the maximum velocity of the wave does not exceed—
being equal , the amplitude of the wave, the masses being in such case rapidly lifted up and let fall again without the withdrawal of the support of the base.
And generally, single objects situated upon the surface of the earth, in firm and rigid connection with it, or so circumstanced that the line of wave transit through the centre of gravity passes through the surfaces of repose and of attachment, move with the earth itself, and are seldom disturbed as to their former position. Thus also, flexible objects, rooted trees, flag staffs, telegraph posts, and the like, are bent by the transverse forces impressed, but return to their positions, leaving only perhaps traces in the earth disturbed at their bases, of the direction of movement.
A few examples may clear this part of the subject. In Fig. I let there be a large stone ball, adherent to a narrow base on top of its pedestal, which is fast in the
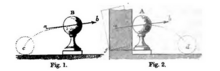
ground, and exposed to a shock, the direction of wave motion of which, in the first semiphase, is from to , and with velocity sufficient to dislodge it. The ball, urged by inertia, and free to fall in any direction, will be projected in to , contrary to the wave, and describing a trajectory in the plane of the wave-path, will fall to , and if its velocity in the horizontal axis be not wholly destroyed (as by falling on soft ground), it may roll, and so cease to give any indication of subsequent value. We are not now concerned with what is the trajectory, or with its modifying conditions. It is obvious that these might be such that the ball shall drop, nearly plumb to the ground, but always in a direction contrary to that of the wave transit.
If a similar ball however, and exposed to a like shock, have a support—such as a wall, for example, at the side a (Fig. 2), which is not overthrown by the shock, but carried along with the wave in the forward movement of its first semiphase—then the ball, although pressed by inertia against the adjacent face of the wall, is prevented falling in that direction. It also, therefore, has impressed upon it, the maximum velocity of the wave in its first semiphase. When, therefore, the wave itself arrives at its maximum velocity in the contrary direction—viz., in its second semiphase—the ball, by its inertia of motion impressed in the previous semiphase, is then thrown in the same direction as the wave transit to ; and projected to the ground at , as before.
It might happen that the wall might be so related in dimensions, &c., to the velocity and direction of the wave, that it should remain standing long enough to produce the effects described upon the ball, but should immediately afterwards begin to fall, fracturing and turning over upon the point in a direction contrary to that of the wave transit; and in such a ease there might remain no evidence to show, from either the ball or the wall, in which direction the wave transit was made, whether from to or the contrary. We might ascertain the path of the wave, or rather the azimuth in which it lay, but no more.
If at two distant localities we can obtain even that much information, we can assign the place of the seismic vertical. For if (Fig. 3) by one shock the ball be projected in the wave-path to , in either direction, suppose contrary to the wave transit, and also the ball at a distant place not in the same right line, also projected, say either as the

former or in the same direction as the wave transit to , then we obtain two azimuths, which can have but one point of intersection in , which is that through which the seismic vertical passes.
Where the body projected (Fig. 4) is circumstanced so as to retain the position in which it alighted upon the ground, so that we can measure the vertical and horizontal axes , , then knowing the maximum velocity of the wave, and which is equal that of projection, we can find the angle of emergence in the, plane of projection whose azimuth is observed, and vice versâ.
And if we have two closely adjacent objects projected in the same locality, and the above conditions observed, we can calculate both the angle of emergence and the velocity. It will most generally happen that a regular solid (such as an obelisk, &c.) will fall prostrate whenever the maximum velocity of the wave is such as to produce in it oscillation sufficient to destroy statical equilibrium; and as the arc of oscillation due to a given velocity may be assigned, if we know the angle of emergence of the wave, so as to arrive at its horizontal component of velocity, we can always assign an inferior limit to the maximum velocity of the wave that overthrew the object whose dimensions, &c., we have observed. And if any other regular solid, although dissimilar in form, can be found at the same locality, which has not been overthrown, we may obtain from it a superior limit of such velocity.
It is possible, however, that oscillation may occur to the limit of equilibrium, or even somewhat beyond it, without involving the fall of the body; for the relation may possibly be such between the time of oscillation of the body (Fig. 5) upon one of its edges or arrises , and the time of a complete phase of the wave, that the equilibrium may be restored by the movement impressed in the second semiphase of the wave in the contrary direction to that first communicated, and before the body has had time to fall over, beyond the limit of such restoration; the adherence or friction of the arris , with the base, producing the necessary hold, by which the wave so acts upon the body during its second semiphase, in the direction to .
If this be sufficient to bring back the centre of gravity through the horizontal distance between the verticals and during the time of the second semiphase of the wave, the body does not fall, but on the cessation of earth movement, topples back to its original position of perpendicularity, overpasses it, and after a succession of decreasing oscillations remains vertical as at first.
In so resuming a position of rest, it may be so circumstanced as to the nature of its base and arrises of oscillation, as to twist considerably from its first position, round one or more vertical axes.
This is a condition of things that very rarely occurs, except with small objects, like vases, statues, or pinnacles consisting of a single block. There are few masses actually found sufficiently hard, when of large size, to prevent the arris splintering or crushing at the first movement to such an extent as to destroy all chance of restoration of position, even if the mass held together as one block; but in walls, towers, campaniles, or other compound masses, made up of blocks more or less firmly united, dislocation at several points takes place from the outset. Such masses being more or less flexible and elastic, bend first, break at the moment of maximum velocity of the wave, and then topple over piecemeal.
With the same velocity of wave, very different effects are produced, with regard to overthrow as the angle of emergence varies, and as the form of the body is different. Thus (Figs. 6 and 7), in the first, the wave emergent in the
direction to , through the centre of gravity produces no disturbance of position in the "boulder stone," the extreme point of the bed preventing rotation in the first semiphase of the wave, by a force measured by , and the point in the second semiphase by a force measured by .
In fact, no velocity of earth wave occurring in nature, even with the emergent angle , i.e., horizontally, could overturn a block proportioned as in Fig. 6. If resting on a bed of earth or stone, it might slide and plough along upon it, and knowing certain coefficients, the length and dimensions of the channel or course cut by it would enable the wave velocity to be arrived at. In the case of the other block (Fig. 7), however, it would be overturned by a wave emergent in the direction to in either semiphase of the wave, the forces of overthrow in each being proportionate to and . So in more regular solids, the column shaft (Fig. 8) may be overturned by a sufficient velocity of wave, in either semiphase, emergent at any angle between , passing through the centre of gravity and the horizontal
wavepath passing through the same, the overthrowing force, with a given emergence and direction of wave-path, to , being proportionate to in the first semiphase and to in the second.
But the pedestal or "cippus" (Fig. 9) can only be overturned in the second semiphase of the wave, however great its velocity, if emergent in the direction to , nor then unless with a very great velocity, the effect of the wave in its first semiphase, however great its velocity, being merely to urge the whole solid against the ground in the direction to ; and if it stand free upon a surface with friction (as an article of furniture, a cabinet or press, for example), to cause it to slide in the direction to horizontally.
The initial velocity of a body projected by earthquake shock, or that of some point of one overturned, is equal to the maximum velocity of the earth wave; for upon the principle of the equality of action and reaction, the greatest effect produced must be due to the greatest applied velocity.
And so also of fractures; they are to be considered as due to a force , being the mass of the fragment broken off, and the velocity of its centre of gravity or of oscillation, and equal to the maximum velocity of the wave, at the instant of its passing through which, fracture occurs.
It will thus be apparent that the principal phenomena presented by the effects of earthquake shock upon the objects usually occurring upon the surface of the inhabited parts of the earth, resolve themselves into problems of three classes, and are all amenable to mechanical treatment, viz.—
- 1st. Problems relating to the directions and amount of velocities producing fracture or fissures.
- 2nd. Problems relating to the single or multiplied oscillations of bodies considered as compound pendulums.
- 3rd. Problems referable to the theory of projectiles;
in which last, as the velocity is small, and the mass usually great in proportion to the range, which is also small, we are not disturbed by any consideration of resistance from the atmosphere.
These three classes of problems frequently are found combined in a single example—thus fracture and overthrow often occur together, or fracture and projection, and sometimes all three are united; a body (a gate pier for example) being broken off at its base, and overturned, but with a velocity more than sufficient for both, so that it is also projected, or thrown to a certain distance from its base. Although a less regular arrangement, it will tend to greater clearness, now to leave the further strict mechanical consideration of these questions, with the preliminary statements that have been made, and proceed first to describe pretty fully, the characteristics and details of structure of the buildings, &c., to which those principles will be applied in the present Report; and then to enter minutely upon the nature. of the fractures or fissures produced by earthquake shock in such buildings, &c, and describe the methods and conditions of observing them, and afterwards treating the observations; and finally to give in a connected form the equations referring to the treatment of all the classes of problems, as respects velocity, or direction obtained by calculation from velocity.
Almost all that follows with reference to the observation of the directions of fissures (or fractures), therefore is to be viewed as descriptive of the methods of arriving at direction only of wave-path, without reference to the velocity of the wave particles, or to any other mechanical conditions except those which determine the directions of such fissures as observed in buildings, velocity being determined, and also emergence, indirectly, by calculation applied to the observed conditions of the forces employed in producing fracture, overthrow, or projection.
Fissures in buildings, not overthrown, are, in fact, the sheet anchor, as respects direction of wave-path to the seismologist in the field.
The observations usually available, upon single or isolated objects, such as pillars, obelisks, vases, or statues overthrown, or others often of small size though comparatively rarer, are generally not less important, as determining direction, than those to be made upon objects of united or complex construction, or however large, such as buildings of various sorts. The latter are, however, the staple indices upon the careful observation of the injuries to which, we are mainly dependent for arriving at the directions in azimuth of shock.