A, we can account for the excess of work done by A over that spent on B solely by supposing that B takes more heat from the condenser than A gives to it. Such a compound engine would transform into work heat taken solely from the condenser. And the work so obtained might be employed on B, so as to make it convey heat to the source while farther cooling the condenser.
Clausius, in 1850, sought to complete the proof by the simple statement that "this contradicts the usual behaviour of heat, which always tends to pass from warmer bodies to colder." Some years later he employed the axiom, "it is impossible for a self-acting machine, unaided by any external agency, to convey heat from one body to another at a higher temperature." W. Thomson, in 1851, employed the axiom, "it is impossible, by means of inanimate material agency, to derive mechanical effect from any portion of matter by cooling it below the temperature of the coldest of the surrounding objects." But he was careful to supplement this by further statements of an extremely guarded character. And rightly so, for Clerk-Maxwell has pointed out that such axioms are, as it were, only accidentally correct, and that the true basis of the second law of thermodynamics lies in the extreme smallness and enormous number of the particles of matter, and in consequence the steadiness of their average behaviour. Had we the means of dealing with the particles individually, we could develop on the large scale what takes place continually on a very minute scale in every mass of gas,—the occasional, but ephemeral, aggregation of warmer particles in one small region and of colder in another.
6. The Laws of Thermodynamics.—I. When equal quantities of mechanical effect are produced by any means whatever from purely thermal sources, or lost in purely thermal effects, equal quantities of heat are put out of existence, or are generated. [To this we may add, after Joule, that in the latitude of Manchester 772 foot-pounds of work are capable of raising the temperature of a pound of water from 50°F. to 51°F. This corresponds to 1390 foot-pounds per centigrade degree, and in metrical units to 425 kilogramme-metres per calorie (see Heat).]
II. If an engine be such that, when it is worked backwards, the physical and mechanical agencies in every part of its motions are all reversed, it produces as much mechanical effect as can be produced by any thermodynamic engine, with the same temperatures of source and refrigerator, from a given quantity of heat.
7. Absolute Temperature.—We have seen that the fraction of the heat supplied to it which a reversible engine can convert into work depends only on the temperatures of the boiler and of the condenser. On this result of Carnot's Sir W. Thomson based his absolute definition of temperature. It is clear that a certain freedom of choice is left, and Thomson endeavoured to preserve as close an agreement as possible between the new scale and that of the air thermometer. Thus the definition ultimately fixed on, after exhaustive experiments, runs:—"The temperatures of two bodies are proportional to the quantities of heat respectively taken in and given out in localities at one temperature and at the other respectively, by a material system subjected to a complete cycle of perfectly reversible thermodynamic operations, and not allowed to part with or take in heat at any other temperature; or, the absolute values of two temperatures are to one another in the proportion of the heat taken in to the heat rejected in a perfect thermodynamic engine, working with a source and refrigerator at the higher and lower of the temperatures respectively."[1] If we now refer again to fig. 1, we see that, and being the absolute temperatures corresponding to PP′ and QQ′, and H, H′ the amounts of heat taken in during the operation PP′ and given out during the operation Q′Q respectively, we have
whatever be the values of and . Also, if heat be measured in terms of work, we have
Thus with a reversible engine working between temperatures and the fraction of the heat supplied which is converted into work is
It is now evident that we can construct Watt's diagram in such a way that the lines of equal temperature and the adiabatics may together intercept a series of equal areas.
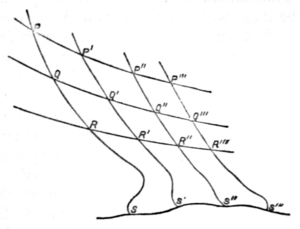
Fig. 2.
Thus let PP′ (fig. 2) be the isothermal , and on it so take points P′, P′′, P′′′, &c., that, as the working substance passes from P to P′, P to P′′, &c., units of heat (the unit being of any assigned value) shall in each case be taken in. Let QQ′, RR′, &c., be other isothermals, so drawn that the successive areas PQ′, QR′, &c., between any two selected adiabatics, may be equal. Then, as it is clear that all the successive areas between each one pair of isothermals are equal (each representing the area ), it follows that all the quadrilateral areas in the figure are equal.
It is now clear that the area included between PP′ and the two adiabatics PQR, P′Q′R′ is essentially finite, being numerically equal to . Thus the temperature for each isothermal is represented by the corresponding area. This is indicated in the cut by the introduction of an arbitrary line SS′, supposed to be the isothermal of absolute zero. The lower parts of the adiabatics also are unknown, so that we may draw them as we please, subject to the condition that the entire areas PS′, P′S′′, P′′S′′′, &c., shall all be equal. To find, on the absolute scale, the numerical values of two definite temperatures, such as the usually employed freezing and boiling points of water, we must therefore find their ratio (that of the heat taken and the heat rejected by a reversible engine working between these temperatures), and assign the number of degrees in the interval.
Thomson and Joule experimentally showed that this ratio is about 1.365. Hence, if we assume (as in the centigrade scale) 100 degrees as the range, the temperatures in question are 274 and 374 nearly. A full discussion of this most important matter will be found under Heat.
8. Entropy.—Just as the lines PP′, QQ′, &c., are characterized by constant temperature along each, so we figure to ourselves a quantity which is characteristic of each adiabatic line,—being constant along it. The equation of last section at once points out such a quantity. If we write for its value along PQ, for P′Q′, we may define thus
From the statements as to the equality of the areas in fig. 2 the reader will see at once that the area bounded by is . We are concerned only with the changes of , not with its actual magnitude, so that any one adiabatic may be chosen as that for which .
9. The Dissipation of Energy.—In the before-cited article Energy (vol. viii. p. 210) this part of the subject has already been treated. Since that article was written Sir William Thomson has introduced the term thermodynamic motivity to signify "the possession the waste of which is
- ↑ Trans. R.S.E., May 1854.