Steam Heating and Ventilation/Chapter VI
Chapter IV.—Piping and Construction Details.
Having selected the system of heating to be employed according to the needs of the building in hand, and having proportioned the radiator surface according to the requirements of the various rooms, it then remains to lay out the system of piping and arrange the various details of construction.
In regard to piping connections, it should be stated at the outset that the flow of steam through any system of piping depends primarily upon the difference in pressure between that at the supply end and that at the delivery or return end, and without any difference of pressure no flow of steam can exist. In exhaust heating or low-pressure gravity systems, this difference of pressure is very slight, and consequently for such systems the pipes have to be larger than for high-pressure heating or vacuum systems in which the pressure in the returns is reduced by connecting them to a vacuum pump. Again, in most systems of steam heating there is another consideration which affects the sizes of pipes; that is, the water of condensation from the radiators. If the steam circulation were uniform and continuous and the water of condensation kept separate from the steam supply, as in properly arranged two-pipe systems, the pipes might be very small; but it is necessary to allow for sudden opening of radiator valves, so as to take care of the momentary demand for steam which this causes, as well as the rush of water of condensation which accompanies it. For exhaust and low-pressure gravity systems, it may be laid down as a general rule that pipe sizes should be larger for the simple one-pipe system than for any other arrangement. They may be smaller for the one-pipe overhead, or Mills system, and still smaller for the two-pipe systems. A description of various systems of steam distribution was given in Chapter II.
Baldwin's rule for pipe sizes.—In the early days of steam heating, pipe sizes were proportioned by various empirical rules, the usual basis of which was the principle of being sure to get the pipes large enough; and such rules are, to a large extent, blindly followed to-day. Mr. William J. Baldwin, in his earlier work on steam heating, gave a rule for proportioning steam pipes which is very convenient and has been very widely used. This rule states that in the sectional area of the pipe there should be allowed the area of a 1-inch pipe for every 100 square feet of radiator surface. Inasmuch as the areas of circles are proportional to the square of their diameters, this means a 1-inch pipe for 100 square feet, 2-inch pipe for 400 square feet, 3-inch pipe for 900 square feet, 8-inch pipe for 6,400 square feet, etc. These sizes are none too large for many cases, although in plants with the system carefully arranged so that the circulation is all in one direction and the water of condensation does not have to flow back against the current of steam, pipes can be very considerably decreased below the sizes given by this rule. Mr. Baldwin also gives a diagram of minimum sizes for short horizontal supply mains from which few branches are taken, which give sizes very much smaller than the rule above quoted. [ See Table I. ]
Mills' rule for pipe sizes.—Mr. J. H. Mills gives a diagram for the sizes of mains for one-pipe overhead systems of which he is the originator. This diagram gives sizes somewhat smaller than those obtained by Mr. Baldwin's rule. In the accompanying table are given the maximum square feet of radiation on pipes of each size according to the rules of Baldwin and Mills, as well as Mr. Baldwin's sizes for return pipes. In regard to his figures for minimum sizes for mains, Mr. Baldwin states that they represent minimum conditions for lengths of 50 feet or thereabout, but that for large buildings one size larger should be used.
Monroe's rule for pipe sizes.—In his own practice the author has divided mains and risers of steam-heating systems into the following classifications:
(a) Supply mains for one-pipe systems, which carry all water of condensation, but in the direction that the steam flows.
TABLE I. PIPE SIZES FOR STEAM HEATING ACCORDING TO BALDWIN AND MILLS. SQUARE FEET OF HEATING SURFACE. | ||||||||
Size of pipe in inches | 1 | 2 | 3 | 4 | 5 | 6 | 8 | 10 |
A. Mills Supply mains and risers |
... | 900 | 1,750 | 2,500 | 3,300 | 4,000 | 7,250 | 10,500 |
B. Baldwin Supply mains and risers |
100 | 400 | 900 | 1,600 | 2,500 | 3,600 | 6,400 | 10,000 |
C. Baldwin Minimum for mains |
... | ... | 1,700 | 3,000 | 5,500 | 8,700 | 16,000 | 22,000 |
D. Baldwin Returns |
... | 1,650 | 3,700 | 6,200 | 10,000 | ... | ... | ... |
(b) Mains for two-pipe or one-pipe overhead systems, into which there is no water of condensation from the radiators.
(c) Supply risers for ordinary one-pipe systems which carry all the water of condensation and in a direction opposite to the flow of steam.
(d) Risers for one-pipe overhead systems which carry all the water of condensation but in the same direction as the flow of steam, the lowest part of riser below last radiator being solely a return pipe.
(e) Supply risers for two-pipe systems which carry no water condensation, except that due to the pipes themselves.
In addition to these the following classification is made for return pipes:
(f) Return mains for two-pipe and overhead systems which are above the water-line of the system.
(g) Horizontal return mains for two and one-pipe systems which are below the water-line.
(h) Return risers for two-pipe systems.
Table II. herewith gives the maximum amount of radiation to be put on each size of pipe for the different classifications:
TABLE II. PIPE SIZES FOR HEATING SYSTEMS (MONROE). | |||||||||
(a) | (b) | (c) | (d) | (e) | (f) | (g) | (h) | ||
Size of pipe, in. | Supply Mains, One-pipe. | Supply Mains, Two-pipe or Overhead. | Supply Risers, One-pipe. | Supply Risers, One-pipe Overhead. | Supply Risers, Two-pipe. | Return Mains, Above Water-line. | Return Mains, Below Water-line. | Return Risers, Two-pipe. | |
1 | 70 | .... | 50 | 60 | 80 | 400 | 400 | 250 | |
1½ | 50 | .... | 120 | 150 | 200 | 900 | 900 | 700 | |
2 | 300 | 400 | 250 | 300 | 400 | 1,600 | 1,600 | 1,200 | |
2½ | 500 | 650 | 400 | 500 | 700 | 2,600 | 3,500 | 2,000 | |
3 | 750 | 1,200 | 700 | 900 | 1,200 | 3,800 | 8,000 | 3,000 | |
4 | 1,400 | 2,000 | 1,500 | 1,800 | 2,000 | 7,000 | 14,000 | .... | |
5 | 2,400 | 3,500 | 2,500 | 3,500 | 3,600 | 12,000 | 26,000 | .... | |
6 | 4,000 | 5,500 | 3,600 | 4,200 | .... | 16,000 | .... | .... | |
7 | 5,500 | 8,000 | .... | .... | .... | 30,000 | .... | .... | |
8 | 7,000 | 12,000 | .... | .... | .... | .... | .... | .... | |
10 | 12,000 | 16,000 | .... | .... | .... | .... | .... | .... | |
12 | 18,000 | 25,000 | .... | .... | .... | .... | .... | .... |
The table here given represents the conditions which are to be met with in ordinary buildings, and exceptional conditions will have to be met with by the judgment of the engineer conducting the work. It will be noted in general that this table gives less radiation on the small pipe sizes and more on the large than that by either Baldwin's or Mills' rules. In small plants, or in plants where a large number of small radiators are supplied from the risers, the number of radiators on a given riser may affect its size irrespective of the amount of radiation. Risers less than 1 inch in size are rarely used on a two-pipe system, or less than 1¼ on an ordinary one-pipe system, unless perhaps for only one small radiator. In an overhead system the height of the building and the consequent number of radiators on the riser affect the size, especially at the lower end. In such a system it must be borne in mind that all the water of condensation from the higher radiators is falling down the pipe, passing the connections to the lower radiators. For such systems in high office buildings it is therefore well to make the risers fairly large toward the bottom while the upper portion can be proportioned according to the sizes given in column (d). For buildings about ten stories high, the lower part of the riser should be not less than 2 inches, and if the amount of radiation on the riser is large or the building is over 15 stories high, this may better be 2½ inches. The table given is intended for low-pressure gravity systems and exhaust heating where not more than 2 or 3 pounds back pressure can be carried on the engine.
For high-pressure systems working at 20 or 30 pounds pressure, such as are used sometimes in factories, when engines are used with a condenser, the pipe sizes may be somewhat smaller than those given in columns (e) and (h), although for the smaller connections it is not advisable to reduce them on account of the possibility of water from the radiators backing into the supply pipes.
Pipes for vacuum systems.—In vacuum systems in which the vacuum is maintained on the return side, pipe connections may be reduced very materially, and Table III, given herewith, shows sizes recommended by Messrs. Warren Webster & Company for mains, risers and radiator connections for the vacuum systems which they install. As already described, their system is in principle an ordinary two-pipe system with a vacuum pump on the returns, but also having the special feature, of an automatic thermostatic valve on the return connection, which valve closes automatically when it is heated to steam temperature and opens when it becomes cooler. From the author's experience he would sider the sizes given in this table somewhat too small and would in general recommend about one pipe size larger.
TABLE III. PIPE SIZES WEBSTER VACUUM SYSTEM. | ||||||||||
Size of supply pipes, in. | ¾ | 1 | 1½ | 2 | 3 | 4 | 5 | 6 | 8 | 10 |
Maximum sq. ft. on runs not over 50 ft. | 100 | 150 | 400 | 900 | 2,000 | 4,000 | 8,00 | 12000 | 30,000 | 60,000 |
Sq. ft. surf. for long runs, 300 to 400 ft. | 40 | 100 | 300 | 600 | 1,500 | 3,000 | 6,000 | 10,000 | 22,000 | 40,000 |
Minimum for return for above, in. | ⅜ | ⅜ | ½ | ¾ | 1 | 1¼ | 1¼ | 1½ | 2 | 2½ |
With vacuum systems which have a vacuum only on the air-valve connection, such as the Paul system, it is impracticable to reduce much the sizes of the steam pipes below those given in Table II, as the only feature of this system is that it keeps pipe and radiators perfectly free from air, and does not greatly affect the flow of steam and water of condensation.
Radiator connections.—The connections from the risers to the radiators are always made somewhat larger in proportion than the mains and risers, and Table IV gives sizes which represent good practice for low-pressure systems.
TABLE IV. RADIATOR CONNECTIONS. | ||||
One-pipe Systems. | Two-Pipe Systems. | |||
in. | Max. surf. in rad. sq. ft. | Supply. in. | Returns. in. | Max. surf. in rad. sq. ft. |
¾ | 25 | ¾ | ½ | 40 |
1 | 50 | 1 | ¾ | 75 |
1¼ | 85 | 1¼ | 1 | 120 |
1½ | 130 | 1½ | 1 | 180 |
Carpenter and Sickles' rule for steam pipe sizes.—In designing piping for large systems it must be borne in mind that there are many things which affect the flow of steam in a piping system, and special cases must have special consideration. Elbows, bends and valves greatly increase friction in the pipes. According to the recent investigation of Professor Carpenter and Mr. E. C. Sickles, as given in a paper before the American Society of Mechanical Engineers, Volume XX, a single 90-degree elbow is equal in frictional resistance to a length of pipe equal to about 520 times the diameter, while the resistance of a globe valve is equal to a length of 706 times the diameter, and a good gate valve does not add any practical resistance. They gave the following approximate formula for diameters of pipes, which they say is practically accurate for sizes over 2½ inches:
in which d equals the diameter in inches; w, the weight of steam, to be delivered in pounds per minute; L, the length of the pipe in feet; D, the density or weight in pounds per cubic foot, and p, the difference in pressure in pounds per square inch between the ends of the pipe. Transposed, this formula becomes:
From this it will be seen that, other things being equal, the delivery is proportional to the square root of the fifth power of the diameter.
The accompanying table, Table V, is calculated from this formula, assuming p = 1 pound per square inch difference of pressure and D = 0.04, which is the density of steam at a pressure of about one pound above the atmosphere. In this table allowance is made also for two globe valves and two elbows to each length of pipe. The square feet of surface each pipe would supply, allowing 0.30 pound of steam per square foot per hour (0.005 pound per minute), which is very liberal for direct radiation, is also given in the table. This table is chiefly interesting when compared with Table II, but may be of value for long mains where the building to be heated is at a distance from the plant. It should be noticed, however, that the greatest resistance is due to the elbows and valves. For example, the 8-inch pipe, 600 feet long, with two elbows and
w = wt. of steam delivered per min. per 1 lb. difference of pressure. | ||||||
R = sq. ft. of radiation supplied at 0.005 lb. per sq. ft. per min. | ||||||
Diameter, in. | Length of pipe allowing for 2 valves and 2 elbows, feet. | |||||
---|---|---|---|---|---|---|
100 | 200 | 400 | 600 | 1000 | ||
3 | w | 8.5 | 7.7 | 6.9 | 6.3 | 5.5 |
3 | R | 1,700 | 1,540 | 1,380 | 1,22 | 1,100 |
4 | w | 15.0 | 14.2 | 12.9 | 12. | 10.6 |
4 | R | 3,000 | 2,840 | 2,580 | 2,400 | 2,120 |
6 | w | . . . . | 33. | 31. | 29. | 26.2 |
6 | R | . . . . | 6,600 | 6,200 | 5,800 | 5,240 |
8 | w | . . . . | 59.5 | 56.5 | 54. | 49. |
8 | R | . . . . | 11,900 | 11,300 | 10,800 | 9,800 |
10 | w | . . . . | 95. | 90.4 | 87. | 81. |
10 | R | . . . . | 19,000 | 18,080 | 17,400 | 16,200 |
12 | w | . . . . | 138. | 132. | 138. | 120. |
12 | R | . . . . | 27,600 | 26,400 | 25,600 | 24,000 |
-addition of another elbow would be equivalent to 350 feet of straight pipe and would reduce the delivery in the ratio of V2,240 -f- 2,590.
Draining pipes. In laying out the piping system for a heating plant, besides the proper size of pipes there are two points which must 'be very carefully considered : (1) That pipes as well as radia- tors are properly drained so that the water of condensation will flow off easily and uniformly to its proper receptacle; and (2) that proper provision be made for the expansion of pipes, so that such expansion shall not interfere with the flow of steam or water or disturb the setting of the radiators.
Pipes for an ordinary one-pipe system, which are run around the basement of a building, should be pitched toward the extreme ends, from which the return connection should be taken and run back to the receiver below the water-line. If the mains are very long they should be drained at intervals into this pipe.
In a two-pipe system in which the mains are similarly run, they should be drained into the return pipes, and in making these drip connections care should be taken that the return pipes into which they drain are lower than the supply mains, so that there will be no opportunity for water to flow from the returns into the supply mains. The return pipes are generally, where possi- ble, run under the basement floor, and should be lower than the supplies. Figures 31 and 32 represent typical connections from mains to risers. Where, on account of economy of space, it is necessary to run the supply and return mains on nearly the same level, as in Figure 32, the supply main must be dripped into a separate pipe run back under the floor or along the wall and con- nected into the principal return main below the water-line of the system. In two-pipe systems where the supply mains are short and properly covered, so that there is not much condensa- tion, they may be given a slight rise from the boiler or source of supply and the water of condensation allowed to flow back. Riser connections should be taken out of the top of the mains so as to prevent any water of condensation that may be in the mains from getting into the risers.
In the one-pipe overhead system there is always a main supply riser running to the branch mains in the attic, and this should have a drain pipe from its lowest point extending back to the receiving tank. The attic mains are drained directly into the supply risers, which drop from the bottom of them.
In connecting up radiators on a one-pipe system they should be set so as to pitch slightly toward the connection from the riser, and the connection should always pitch toward the riser. Con- nections which, on account of carelessness in workmanship, were pitched in the opposite way, are a very fertile cause of water- hammer. Eadiators set on two-pipe systems should be pitched slightly toward the return connection, which should not be con- nected from the same end as the supply. There are a number of plants in which radiators connected on two-pipe systems have both the supply and return connections at the same end of the radiator, but in the opinion of the author this is a very bad prac- tice, as the radiator might very much better be connected on the one-pipe system. In fact, when radiators with such connections are in operation, unless the return connection is lower than the supply, which is not generally the case, the water of condensation is just as apt to Tun down the supply connection as down the re- turn. Furthermore, when such radiators are turned on, if the sup- ply valve is opened first, any water which may be in the radiator runs out of this connection, as well as the large amount that is formed by the first contact of steam with the cold radiator; and if the return valve is opened first, the water in the return pipe backs up into the radiator, so that when the supply valve is opened, a large amount of it will run out to the supply connec- tion. At this point it should be stated that in turning on radia- tors with two-pipe connections the supply valve should always be opened first; and the fact that the uninformed occupants of rooms frequently do not know which is the supply valve is one of the objections of two-pipe systems.
Expansion of pipes. The expansion of pipes is an important consideration in any case, and where there are long mains or in high office buildings, which consequently have long vertical risers, it becomes a consideration of vital importance. The coefficient of expansion of wrought-iron pipe is 0.000007 per degree Fahr. This amounts to about 1.5 inches in a 100-foot length for low-pressure steam pipes. In horizontal mains this can be gen- erally taken care of by making turns or offsets in the mains in every 50 or 75 feet of pipe, the expansion being taken up by the spring of the pipe. All connections from mains or risers should be made with sufficient length of horizontal connection to allow for this expansion. In Figures 31 and 32 the expansion of the mains and risers is taken up in the spring of the arms, AB. An old rule for the length of such expansion arms is that the length in feet should be equal to twice the diameter in inches. This, is a fair rule in most cases, but much depends on the amount of expansion to be taken care of, and no set rule can be given.
The most serious difficulties on account of expansion are met with in the long vertical risers of the modern high office buildings. In such cases any considerable movement of the riser is apt to result in trouble, as the radiator connections are generally short.

Fig. 31

Fig. 32
Provisions for Drainage and Expansion of Piping.
Various means are employed to overcome this. In buildings over ten stories in height it can generally be taken care of by anchoring the risers rigidly in the middle so they expand in both directions, and allowing for the expansion, by the connections to the supply main in the attic and to the returns in the basement. The radiators on the upper and lower floors, where most of the expansion takes place, must have connections sufficiently long to allow for it, and they must have sufficient pitch, so that they will not be trapped by the expansion of the risers. The author is familiar with one building 14 stories high in which expansion is entirely taken care of in this way. Radiators on the extreme floors are made with extra high legs, and the connection from riser isas shown in Figure 33. In another case, in a 14-story building, an offset was made in each riser over the windows of the seventh floor, the upper part running on the opposite side of the tier of windows from the lower part, the spring of the pipe in this offset taking care of the expansion at this, point. The risers were anchored rigidly in the center of each section. Arrangements of this kind are frequently used, and the chief objection is that unless they are concealed the offsets make an unsightly appearance, and it is frequently very inconvenient to put them in on account of the arrangement of the building.

Fig. 33

Fig. 34

Figure 36.—Piping in Attic of Ellicott Square Building, Buffalo.
Figure 33. If the radiator connections were very short, two joints would have been put on each of these risers.
In laying out large systems, valves should be placed on each riser so that each one can be shut off independently of the others in case of leaks or in case of repairs or changes to be made on any of the radiators. Gate valves should be used preferably on account of the fact that they interpose infinitely less resistance to the flow of steam or water than do globe valves. Furthermore, in such cases provision should be made for changes in the arrange- ment of rooms and consequent changes in the location of radiators. It is a very good practice to put tees on each riser at each floor whether or not, in the first instance, a radiator connection is re- quired, as subsequent changes in the arrangement of rooms may make it desirable to change the radiators.
Figure 36 shows the arrangement of piping in the attic of the Ellicott Square, a large 10-story building in Buffalo, N. Y., which is heated by direct radiation on the overhead system. The figure illustrates the method of connecting the overhead mains to the risers, and also the way in which expansion of the mains is pro- Tided for by bends and offsets. In this instance the piping was rigidly anchored at four points marked D, and the expansion al- lowed in all directions from these four points. It may be noted here that branch mains were taken off at points marked E and F, instead of connecting each riser to the main 10-inch pipe at these points. This was done for the purpose of saving the expense and 'the delay to the work of connecting each riser into the 10-inch pipe.
Valves. Much care should be used in placing valves on a piping system. Gate valves should always be used on mains. If globe valves are used anywhere, the stems must be placed horizontally, as otherwise they form a water pocket. Thermostatic valves, so- called, are often used on radiators, being connected with an auto- matic device which opens the valve when the temperature falls, -and closes when it rises.
Location of risers. In laying out the floor plans for the heating system of a large office building it is a mistake to try to reduce the number of risers to a minimum. It is much better to put in risers enough so that a radiator can be placed under any window in the building without too long a connection from the riser, for in such buildings one can never know what changes in the arrangement of rooms or in the location of radiators may ultimately be desired. Figure 37 shows the typical floor plan of the heating diagrams for a fourteen-story building in Chicago, showing the location of risers and radiators. This building is exceptional on account of the large number of bay windows and large amount of glass surface. Furthermore, the risers were all concealed in the columns in the manner shown in Figures 38 and 39, the building being framed with Gray columns, built as indicated. An expan-

Figure 37.—Plan Showing Location of Risers and Radiators.
sion joint was placed on each riser, above the radiator connection at the eighth floor, with flange unions above and below the joint. At these joints a removable wooden panel was placed over each riser, as indicated at Figure 38, but otherwise they were enclosed by the wire-lath and plaster forming the ordinary finish of the columns. Figure 39 shows a section of the column at the fourteenth floor. The radiator connections were exposed above the floor and run about as indicated on the floor plan. This building is heated on the one-pipe overhead system. It contains 11,000 square feet of radiation, supplied by an 8-inch main to the attic. The typical floor has 1,055 square feet of glass surface, 2,025 square feet of wall surface and 47,400 cubic feet of space, including corridors, and is heated by 18 radiators containing 787 square feet of surface. By the author's formula given on page 68 the amount of surface required amounts to 730 square feet, but the exposure of the upper stories of this building is unusually severe.
It is frequently a very difficult matter to conceal risers in fireproof buildings on account of the floor plates of the columns and the beams, which frequently interfere with placing the risers very close to them. Figure 40, however, represents the manner in which they were enclosed in a building which was framed with box columns. In this case the tile fireproofing was put on over both column and riser. In concealing risers in the walls of wooden buildings it is necessary to protect the pipes carefully from immediate contact with the woodwork. In hanging risers in buildings

Methods of Running Risers in Columns.
great care must be taken that the pipe be cut to the proper lengths so that the fittings for the radiator connections will come exactly in the proper place.
Riser anchors.—As previously stated, risers are usually, especially in large buildings, anchored rigidly at certain points so that expansion shall be in both directions from these points. This should be carefully done so that the pipe will not slip, and the method to be employed to accomplish this depends largely upon the local conditions. Figures 41 and 42 show two methods of accomplishing this, the latter being especially adaptable where the riser can be run close to the floor beam, but to make it perfectly rigid it should be made strong and shrunk in place. The method indicated by Figure 41 can be adapted to anchoring the pipe to a column instead of to the floor beams. In some cases risers are also secured at the other floors so as to allow expansion, but at the same time maintain proper alignment; but this is not generally necessary, as the rigidity of the piping and connections is generally sufficient to keep the pipes property in line.
Protecting pipes.—Where risers or other pipes run through the floors or walls they are generally protected by floor sleeves with floor and ceiling plates. These are usually made of galvanized iron in a telescopic form so as to fit any thickness of floor. In buildings with wooden floors they are necessary so as to give an air space around the pipe and prevent immediate contact of the steam pipe with the woodwork. In fireproof buildings they are
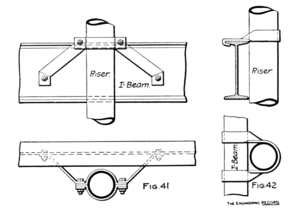
Types of Riser Anchors.
frequently omitted, but it is preferable to use them, as they make a better finish around the pipe at the ceiling and prevent the expansion of the pipe from disturbing the flooring or plaster. Floor and ceiling plates should be used in any case.
Radiator connections are frequently encased in the floor, but it is generally difficult to accomplish this in fireproof buildings, as the space between the floor level and the top of the iron beams is not generally sufficient to box in the connections and make proper allowance for the vertical movement of these connections due to riser expansion. It can be done in some cases, however, but the connections should always be enclosed in a galvanized-iron box and the flooring should be so laid that a strip over the pipes can be easily removed.
Supporting pipes.—Horizontal pipes are almost invariably supported from the ceiling above by means of some kind of an expansion hanger, two common types of which are shown in Figures 43 and 44, the two shown in each case being, one for wooden beams and the other for iron. The rods can be cut to the length desired after the pipe is in place. There is sufficient movement of the rod at the top to allow for the small play of the pipe due to expansion. A simple and cheap form of hanger frequently used for small pipes in buildings with wooden floors is made of a piece of light chain looped under the pipe and hung from the nails in the floor beams. The chain can be cut to length with wire nippers. In case of very large pipes in the basement of buildings, they are

Expansion Pipe Hangers.
sometimes supported by some kind of a standard erected from the floor.
Arrangement of pipes.—In laying out the main piping connections of the power plant of a large building great care must be taken to arrange the pipes as systematically as possible so that they take up no more room than necessary, and also to properly provide for the drainage of all pipes into proper receptacles. This is frequently a difficult matter, but one can hardly give too much consideration to the subject, as the successful operation of a plant depends largely upon the way piping connections are arranged. It is impossible to give any detailed rules, as each plant is a prob
Figure 45.—Piping in an Office Building Plant.
Figure 45 shows the arrangement of the main piping connections in a large office building in Syracuse, N. Y., in which, on account of the extreme difference in floor levels and the crowded condition of the machinery, a really systematic arrangement of piping it was impossible to obtain. (The Engineering Record of November 5, 1898.) The main valves controlling the heating sys-

tern are indicated at A, B, C and D. During the heating season the valve, D, is opened and the back-pressure and reducing-pressure valves put into service, while during the summer months the valve, D, is closed entirely, shutting off the heating mains, and the 10-inch back-pressure valve is opened wide. Ordinarily, both in winter and summer, the valve, A, is closed, so that all exhaust steam from the pumps and engines goes through the muffler tank and heater; but in case it is necessary to' open these for cleaning, the valves B and C are closed and the valve, A, opened, so that the exhaust steam may go directly either into the free exhaust or the heating system, as the case may be. The building in question contains about 15,000 square feet of radiators and is heated on a two-pipe system with basement mains. The returns come back to the two automatic governors which control the 6x4x6inch pumps. These deliver the return water through the closed heater into the boilers. There are three pumps used for this pur- pose, and so connected that any one can be used on the governors separately or together, and any one can be used to pump cold water through the heater. The feed pipe has a by-pass around the heater, to be used when the heater is being cleaned.
Return pipes should be given as much pitch as possible except where they are below the water-line of the system. In running these below basement floors they should be put in trenches, prefer- ably of brick or concrete, and with movable covers. If there is danger of water underground the trenches must be arranged so that they can be kept dry, and no better trench can be made than one of good concrete.
Pipe coverings. All the piping connections should, as far as. possible, be covered with some kind of non-conducting pipe cov- ering, of which there are innumerable varieties made. In some cases risers and other pipes are left uncovered so as to utilize the- heating effect, but the disadvantage of this is that heat is given out from such pipes whether it is wanted or not, and it is much better practice to cover the risers and depend on the radiators, for heating. One of the greatest sources of fuel waste is found in uncovered mains in basements of buildings where heat is noth- ing but an inconvenience, and in order to dispel it in moderate weather windows are opened, which greatly increase the wasteful condensation. A good pipe covering will save from 65 to 80 per cent, of the heat which would ordinarily be wasted from the pipes. Coverings of which 85 per cent, is carbonate of magnesia, certain molded forms of pure asbestos fiber, and molded forms of mineral wool are the best kinds of protection for steam pipes to reduce condensation. Some coverings which show very good results when new, deteriorate rapidly, due to the charring effect of the pipes and to disintegration.
Pipes and flues for indirect radiators. "We come now to the con- sideration of certain details of construction which are especially requisite in indirect heating. In this class of steam heating the piping connections are subject to much the same rules for run- ning pipes as those for direct radiators, but indirects are almost invariably located in the basement of buildings and the pipes run- ning to them are horizontal. Furthermore, the condensation per square foot of indirect radiator is from 25 to 50 per cent, more than that per square foot of direct radiator, so that the piping connections are generally made about a size larger, and, except in rare cases, connected on two-pipe systems. Indirect radiators are usually hung from the beams of the first floor, and various methods, which are dependent upon the local conditions, are adopted for supporting them. A frequent form of support is indicated in Figure 46, the radiator resting on short pieces of pipe which are hung by rods bolted to the floor joists, or hung from a pipe over them. Indirect radiators are always encased in some kind of a metal box, either of galvanized iron or tin, or of wood lined with tin. These boxes connect directly with the hot-air flues which run to the rooms above and which are of heavy tin or sheet iron. Both the flues and boxes should, of course, be as nearly air tight as possible. In regard to sizes of hot-air flues, an

Figure 46.—Indirect Radiator Support.
old rule gives 1 square inch of flue area to 1 square foot of radiator. This is very satisfactory in most cases, but the following table, which gives the sizes recommended by Prof. J. H. Kinealy, is to be preferred, as the size of flue should depend upon its height:
Height in feet from center radiator to center of register | 5 | 10 | 15 | 20 | 25 |
Sq. in. of flue area for 1 sq. ft. radiation | 1.7 | 1.2 | 1.0 | 0.85 | 0.75 |
The indirect-radiator boxes must, of course, have a fresh-air inlet. This should always be run from the outside and from a location removed from the possibility of contamination to the incoming air; and it is preferable that the cold-air inlet be located at least a few feet above the ground. Cold-air supply connections from the outside to indirect boxes should be made as short as possible; 1 square inch area per 1 square foot is generally sufficient, although if the flues are of considerable length or are winding, a larger ratio should be given. If a number of radiators receive air from the same cold-air flue, the flue may be somewhat smaller.

Figure 47.—The Indirect System in a Massachusetts Residence.
In buildings in which there are a considerable number of indirect radiators there are two general methods of connecting the fresh-air flues, which are illustrated in Figures 47 and 48. Figure 47 represents the cellar plan of a large Massachusetts residence (The Engineering Record, August 5, 1893; Mr. A. A. Sanborn, Boston, heating contractor), in which there are seven large clusters of indirect radiators which supply about 30 hot-air flues rising to the rooms above, the hot-air pipes running horizontally from the radiator boxes, in some cases for 50 feet, to the vertical flues. radiators in this are all Gold's Pin in 16-foot sections.) In the Philadelphia residence shown in Figure 48 (The Engineering Record, December 15, 1894), there is, on the contrary, a long main cold-air duct which supplies a large number of indirect radiators, one for each of the vertical flues, the radiator in all cases being located directly under the vertical flues. In the opinion of the author this is much the preferable method, as a much more positive circulation of air to the separate rooms can be secured than by the other method.
The system shown in Figure 48 is interesting also on account of the construction of the main cold-air duct and the connections from it to the radiator boxes. These are well illustrated in Figure 49, the main duct, it will be noted, being of brick, and underground. In the author's opinion there is one particular in which the system shown in Figure 48 might have been much improved. The cold-air duct is long and winding, and had it been more uniform in size

Figure 48.—The Indirect System in a Philadelphia Residence.
and supplied with another cold-air connection on the side of house opposite the existing one, it would have insured a more positive circulation to all the radiators. The reason of this is that in cases, such as shown in Figure 48, where there is only one cold-air connection for a number of radiators, when there is a strong wind blowing against the side of the house opposite the fresh-air inlet it is sometimes very difficult to get a good draft in the flues, especially in those most removed from the cold-air inlet, as the force of the wind (which, with the best constructed houses, blows through the walls to a great extent), seriously opposes the current of air in the ducts. If there are two fresh-air connections, each provided with dampers, the one on the leeward side of the building can be closed and the one on the windward side opened to give a proper amount of cold air. It is, moreover, desirable to put a tight damper in the duct to each radiator.
Setting direct-indirect radiators.—In regard to the setting of direct-indirect radiators, the piping connections are made according to precisely the same rule as for directs, although in cases of large radiators of this kind it may be desirable to increase slightly the pipe sizes on account of the somewhat increased condensation. A

frequent form of fresh-air connection for this kind of radiator is indicated in Figure 50, and the connection to the outside air should in all cases be provided with an easily adjusted damper. One trouble with direct-indirect radiators is that when a strong wind is blowing against the outside wall it is difficult to prevent objectionable drafts, due to sudden gusts of wind, which, in cold weather, will make frequent cold waves across a room notwithstanding the average temperature may be about right. Figure 51 represents a special form of setting for large radiators of this kind adopted by Mr. Alfred R. Wolff, in the Singer Building, New York City. (The Engineering Record, September 3, 1898.) It will be seen that the effect mentioned is here avoided by making the cold-air inlet to the radiator somewhat tortuous, so that, as far as possible, the draft is due only to the hot air from the radiator. The same effect is accomplished in the United States Government method
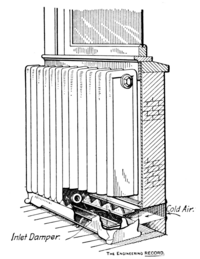
Figure 50.—A Direct-Indirect Radiator.

Types of Direct-Indirect Radiator Casings.
of setting indirects in the Detroit Post Office, which is shown in Figure 52. Mr. Henry Adams, of Baltimore, Md., was the engineer for this work. (The Engineering Record, August 7, 1897.)